This post may contain affiliate links. If you make a purchase through these links, we may earn a commission at no additional cost to you.
Your brain is constantly interpreting the visual world around you, making split-second decisions about what you’re seeing based on incomplete information. This remarkable ability usually serves you well—except when it doesn’t. Optical illusions exploit the shortcuts and assumptions your visual system makes, creating a fascinating gap between physical reality and your perception of it.
While scientists have made tremendous progress in understanding human vision, certain visual phenomena continue to baffle researchers despite decades of investigation. These persistent mysteries entertain us and provide valuable windows into the inner workings of consciousness itself.
This article explores five particularly mesmerizing optical illusions that continue to challenge our scientific understanding of perception. These visual puzzles reveal the boundaries of current psychological and neuroscientific knowledge, demonstrating just how much we still have to learn about how we see the world.
Understanding Optical Illusions
Before diving into specific examples, it’s helpful to understand what optical illusions are and why they occur. Visual illusions arise from the complex relationship between your eyes and brain. Your visual system doesn’t passively record the world like a camera; instead, it actively constructs your perception based on incoming sensory data, prior experiences, and evolutionary adaptations.
Psychologists typically categorize optical illusions into three main types:
Physiological illusions result from excessive stimulation of specific neural pathways (brightness, color, motion, etc.). These illusions directly affect the eyes or visual system through properties like brightness, tilt, or movement.
Cognitive illusions occur when your visual perception is impacted by unconscious inferences. Your brain makes assumptions based on rules it has learned about the physical world, sometimes leading to misinterpretations.
Literal illusions involve images that create figures that differ from the objects that make them up, such as ambiguous or impossible figures.
The value of studying these visual tricks extends far beyond mere entertainment. Illusions serve as critical tools for understanding consciousness, revealing how your brain constructs reality rather than simply recording it. They highlight the distinction between sensation (what your eyes detect) and perception (what your brain interprets), providing researchers with powerful methods for investigating neural mechanisms that would otherwise remain hidden.
With this foundation established, let’s explore five remarkable illusions that continue to resist complete scientific explanation.
1: The Spinning Dancer Illusion
First published by web designer Nobuyuki Kayahara in 2003, the Spinning Dancer (also known as the silhouette illusion) features the shadowy outline of a dancer spinning on one leg. The fascinating aspect of this illusion lies in its bistable nature—viewers typically perceive the dancer rotating either clockwise or counterclockwise, and can sometimes switch between these interpretations.
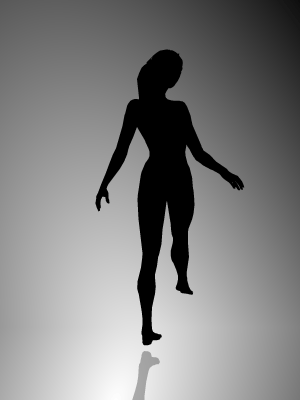
This illusion quickly went viral as people debated the “correct” direction of rotation, with some claims suggesting that the perceived direction revealed which brain hemisphere (left or right) was dominant in the viewer. However, this popular interpretation oversimplifies the complex reality of this visual puzzle.
What makes it mysterious: While scientists understand that the illusion works because the silhouette lacks visual depth cues, they still cannot fully explain several aspects of this phenomenon, including:
- Why some people can switch perspectives easily while others remain “stuck” seeing rotation in only one direction
- The neurological mechanisms that trigger perspective shifts when they do occur
- The relationship between attention, expectation, and perceptual switching
Research on the Spinning Dancer continues to challenge our understanding of visual processing pathways. Studies using functional MRI have shown that perceptual reversals activate areas associated with higher cognitive functions rather than just primary visual areas, suggesting this illusion involves complex interaction between bottom-up sensory information and top-down interpretive processes.
The illusion also reveals fascinating individual differences in perception. Some viewers report being able to control the direction of rotation through conscious effort, while others find their perception shifts unexpectedly or remains fixed despite attempts to see the alternative. These variations hint at underlying differences in visual processing that remain poorly understood.
Current theories suggest that without clear depth cues, your visual system makes an educated guess about the figure’s orientation in three-dimensional space. However, the exact mechanisms determining which interpretation dominates—and when and why perception switches—remain active areas of research without definitive answers.
2: The Hermann Grid Illusion
Discovered by Ludimar Hermann in 1870, the Hermann Grid consists of a simple arrangement of black squares on a white background, creating the appearance of a grid with white lines intersecting at right angles. The illusion occurs when dark spots appear to flicker at the intersections of the white lines as you look around the grid—but when you try to look directly at any particular intersection, the dark spot disappears.
By Tó campos1 – Own work, Public Domain, LinkFor over a century, the standard explanation for this effect involved lateral inhibition—a neural process where excited retinal cells inhibit the activity of adjacent cells. According to this theory, receptive fields at intersection points receive inhibition from all four sides, while those along straight lines experience inhibition from only two sides, creating the illusory dark spots.
What makes it mysterious: Despite the widespread acceptance of the lateral inhibition theory, recent research has revealed significant problems with this explanation:
- The illusion persists when the grid lines are curved or distorted, which shouldn’t happen according to the classical explanation
- The effect weakens or disappears when the grid is rotated 45 degrees, something not predicted by simple lateral inhibition
- The illusion is significantly reduced when white dots are placed at the intersections, which lateral inhibition cannot explain
In 1999, neurophysiologists Baumgartner and Levine proposed an alternative explanation based on S1 simple cells in the visual cortex rather than retinal cells. However, even this updated theory fails to account for all observed properties of the illusion.
Modern computational researchers like Jacques Ninio have suggested that the effect might involve more complex “contour detection” mechanisms in the visual system or possibly even higher-level cognitive processes rather than simply low-level retinal effects.
The Hermann Grid illusion challenges our understanding of basic visual processing and demonstrates how even seemingly simple visual phenomena can involve multiple levels of neural computation. Despite being one of the oldest documented illusions, a complete and satisfactory explanation continues to elude scientists, highlighting the complexity of even foundational visual processes.
3: The McCollough Effect
Discovered by Celeste McCollough in 1965, this remarkable illusion demonstrates a curious relationship between color and pattern perception. The effect is produced by staring at colored grid patterns (typically red and black vertical lines alternated with green and black horizontal lines) for several minutes. After this “adaptation” period, when viewing plain black and white grids, observers perceive the vertical grid as having a greenish tint and the horizontal grid as having a pinkish tint—the complementary colors to those used during adaptation.
By Grid_for_McCollough_effect.png: Android Mousederivative work: Urutseg (talk) – Grid_for_McCollough_effect.png, Public Domain, LinkWhat makes the McCollough Effect particularly fascinating is its extraordinary persistence. While most aftereffects fade within seconds or minutes, this effect can last for hours, days, or even weeks after just a few minutes of initial exposure.
What makes it mysterious: The McCollough Effect defies simple explanation for several reasons:
- Its remarkable longevity suggests it cannot be explained by simple neural fatigue or adaptation
- The effect is orientation-specific, linking color perception with pattern recognition in ways not fully understood
- It shows interocular transfer (training one eye can affect perception in the other) but with reduced strength, suggesting both monocular and binocular neural mechanisms are involved
- The effect demonstrates properties of both neural adaptation and associative learning, blurring the line between sensory adjustment and memory
Current theories fall into two main camps. Some researchers view the effect as an adaptation of orientation-selective neurons that also process color in the visual cortex. Others propose it represents a form of associative learning or conditioning, where the visual system learns to associate specific orientations with particular colors.
Neuroimaging studies have implicated early visual areas like V1 and V2 in the effect, but the precise neural circuits and mechanisms remain unclear. The McCollough Effect continues to challenge conventional categories in vision science, existing at the intersection of adaptation, learning, memory, and perception.
The mystery deepens when considering individual differences in susceptibility and duration. Some people experience the effect strongly for days, while others see it weakly for just hours. These variations suggest genetic or developmental factors in visual processing that we’re only beginning to understand.
4: The Impossible Trident (Blivet)
The Impossible Trident (also known as the blivet, poiuyt, or devil’s fork) presents viewers with a seemingly normal three-pronged fork at one end that impossibly transforms into a two-pronged fork at the other. First appearing in the March 1965 issue of Mad magazine, this figure belongs to a class of “impossible objects” that cannot exist in three-dimensional reality despite appearing plausible at first glance.
By AnonMoos – This vector image includes elements that have been taken or adapted from this file:, Public Domain, LinkWhen viewing the Impossible Trident, your visual system struggles to reconcile contradictory visual cues. Each local section of the figure makes sense, but the global structure violates the rules of three-dimensional geometry. Your brain attempts to construct a coherent 3D mental model but fails due to the inherent contradictions in the image.
What makes it mysterious: The psychological mechanisms behind impossible figures reveal fascinating aspects of visual processing that continue to puzzle researchers:
- The precise point at which the visual system detects the impossibility varies significantly between individuals
- The neural basis for the discomfort or fascination these figures produce remains poorly understood
- The relationship between 2D interpretation and 3D construction in the brain is more complex than previously thought
- Impossible figures expose fundamental questions about where perception ends and cognition begins
Current theories suggest impossible figures activate conflicting neural populations representing different interpretations of depth and spatial relationships. Functional MRI studies have shown increased activity in both the visual cortex and higher-level problem-solving areas when viewing these figures, indicating a complex interplay between perceptual and cognitive processes.
The Impossible Trident challenges our understanding of object recognition and visual integration. While we know the illusion involves conflicts between local and global processing, science hasn’t fully mapped the neural circuitry responsible for detecting these inconsistencies or explained why some people experience stronger reactions to impossible objects than others.
The study of impossible figures continues to provide valuable insights into how the brain attempts to construct coherent reality from visual input, but a comprehensive neurological explanation remains elusive.
5: The Motion Aftereffect (Waterfall Illusion)
The Motion Aftereffect (MAE), sometimes called the Waterfall Illusion, occurs after viewing a moving stimulus for an extended period. When you then look at a stationary scene, it appears to move in the opposite direction. This effect was first noted by Aristotle but received its common name from Robert Addams, who described the phenomenon in 1834 after staring at a waterfall and then shifting his gaze to the rocky cliff beside it.
Paulnasca at English Wikipedia, Public domain, via Wikimedia CommonsTo experience this illusion, stare at a moving stimulus like a spinning spiral or scrolling stripes for about 30 seconds, then look at a stationary object. The stationary object will appear to drift in the opposite direction of the original motion.
What makes it mysterious: While scientists have a general understanding that the effect involves neural adaptation, several aspects remain incompletely explained:
- The duration of the aftereffect varies widely between individuals and conditions in ways that aren’t fully predictable
- The effect sometimes transfers between eyes (looking with one eye and testing with the other), suggesting higher visual processing beyond simple retinal adaptation
- Cognitive factors like attention can modulate the strength of the effect, indicating complex interactions between perception and higher brain functions
- The aftereffect can be contingent on specific properties like color or pattern, revealing specialized neural channels whose interactions aren’t completely mapped
The traditional explanation involves the adaptation of motion-sensitive neurons in the middle temporal visual area (MT/V5). According to this theory, neurons that detect motion in a particular direction become fatigued, temporarily reducing their responsiveness. When you then view a stationary object, the reduced activity in these neurons creates an imbalance that produces the illusion of motion in the opposite direction.
However, this simple model fails to account for the full complexity of the phenomenon. Recent research suggests the aftereffect might involve predictive processing mechanisms, with the brain actively recalibrating its expectations about motion rather than simply experiencing neural fatigue.
The Motion Aftereffect provides a window into the dynamic nature of visual processing, demonstrating how your perception continually adjusts to environmental conditions. Despite being one of the oldest documented illusions, scientists continue to debate its complete neural basis, making it a enduring mystery in vision science.
The Psychology Behind the Mystery
Looking across these five illusions, several common themes emerge that help explain why they continue to resist complete scientific explanation.
First, these phenomena expose the multi-level nature of visual processing. Vision doesn’t happen in a single area or through a simple pathway—it involves parallel processing across dozens of specialized brain regions, from the retina through the thalamus and multiple cortical areas. Our scientific understanding of these complex interactions remains incomplete, particularly regarding how different visual properties (motion, color, form, depth) integrate to create unified perception.
Second, these illusions highlight the challenge of connecting subjective experience to neural activity. While neuroimaging technologies like fMRI, EEG, and MEG provide valuable insights into brain activity during illusions, they measure correlates of perception rather than perception itself. The explanatory gap between neural firing patterns and conscious experience—sometimes called the “hard problem” of consciousness—remains a fundamental challenge.
Third, individual differences in susceptibility to illusions suggest genetic, developmental, and experiential factors that shape visual processing. The variations in how people experience these phenomena remind us that perception isn’t universal—each person’s visual system has been uniquely shaped by their life history in ways difficult to fully account for in scientific models.
Perhaps most importantly, these stubborn mysteries demonstrate the limits of reductionist approaches to understanding perception. Vision emerges from the complex interaction of bottom-up sensory processing and top-down influences like expectation, attention, and prior knowledge. This bidirectional flow of information creates emergent properties that can’t be fully explained by examining individual components in isolation.
As computational neuroscientist Bruno Olshausen noted, “We know far more about how the retina works than about how visual cortex works, and far more about how visual cortex works than about how the mind works.” These illusions occupy the fascinating boundary zones between these levels of understanding.
Conclusion
The five optical illusions explored in this article—the Spinning Dancer, Hermann Grid, McCollough Effect, Impossible Trident, and Motion Aftereffect—continue to challenge our scientific understanding of visual perception. While researchers have developed partial explanations for each phenomenon, complete accounts remain elusive despite decades of investigation.
These persistent mysteries remind us that perception is not passive reception but active construction. Your visual experience emerges from complex interactions between sensory input, neural architecture, past experiences, and evolutionary adaptations. The gaps in our understanding highlight how much remains to be discovered about the relationship between brain activity and conscious experience.
Far from being mere curiosities, these illusions serve as powerful research tools. By exposing the limits and mechanisms of perception, they provide valuable insights into how we construct our visual reality. As neuroscience and psychology continue to advance, these phenomena help map the boundaries of current knowledge while pointing toward new avenues of investigation.
Perhaps the most profound lesson these visual puzzles offer is an appreciation for the remarkable complexity of ordinary seeing. The next time you open your eyes, remember that your seamless visual experience represents an extraordinary feat of neural computation—one whose inner workings continue to fascinate and challenge even our most sophisticated scientific understanding.
Try It Yourself
Experience these fascinating illusions firsthand to observe how your own visual system responds. Notice any individual quirks in your perception—can you easily switch perspectives in the Spinning Dancer? How long does the McCollough Effect last for you? These personal observations connect you to the ongoing scientific exploration of human perception, reminding us that some of the most profound mysteries exist not in distant galaxies but in the everyday miracle of how we see the world.